Background science for the Turkish mutations, recap and III.
An influenza virus does only one thing: tries to make many copies of itself. And it does it poorly, although prolifically. Instead of making exact copies it is liable to make inexact copies and this is one of the sources of genetic variation which produces the parallel "random experiments" characteristic of viral replication. Lots of the copies it makes are fine, but lots are fatally flawed, and there are also many that are more or less imperfect copies but which might have properties that turn out to be useful if the environment changes, for example, if the host makes antibodies to the original version or the virus finds itself in a colder, warmer or unfamiliar host. One thing is certain, however. The virus cannot replicate without infecting a host cell. Viruses, unlike bacteria, plants or animals, are not "free living" forms. They have to hijack a living cell and commandeer its copying machine and protein workshop to make more copies of itself. To do this it has to get into a living cell. In the first two posts of this series we have been discussing a tiny slice of the replication process: how a virus finds a cell to hijack (infect).
We know that viruses aren't completely promiscuous, however. They usually are restricted in the kinds of animals and the tissues or organ cells they infect. In humans, influenza is primarily (although not exclusively) a respiratory disease, at least at first. Moreover, influenza viruses that infect birds don't usually readily infect humans. We now know that influenza viruses can "jump the species barrier" and ones that formerly just infected birds can start infecting humans. Since H5N1 is primarily a bird (avian) influenza virus, and in birds usually infects the bird's intestines, the question of what allows a virus to infect particular animals and tissues is obviously an important question.
In Part I of this series we discussed what the influenza virus "sees" when it cruises by a cell, describing the lawn or canopy of sugars that cover the surface of most cells. Part I. also introduced some terms (like glycolipid and glycoprotein) you will encounter when reading papers about influenza. The main idea, however, was to give you a picture (both literally and conceptually) of where the action is taking place at the surface of a host cell. In Part II I discussed a particular sugar that tips the ends of this lawn of sugar chains sticking up from the cell's surface. It is called sialic acid. The terminology that is used in this field is keyed to the details of the chemical structure of sialic acid, so we spent most of Part II explaining that terminology. It isn't really necessary to know why something is called an α-2, 3 linkage or an α-2, 6 linkage, but I like to know these things and I thought I'd take the time to explain it to you. My (long) experience as a professor has taught me that people get pleasure out of understanding things, even if it is only vaguely. If it's not your cup of tea, just skip it.
Here's a quick summary of the gist of Part II. The particular sialic acid we are concerned with has a modification to it, an attached chemical group called an acetyl group, hooked on at the #5 carbon through a Nitrogen atom. We show the numbering and other stuff in the post. Sialic acid also has another name, neuraminic acid, so this modified sialic acid is also designated Neu5Ac (neuraminic acid N-acetylated at position 5; somehow the "N" part didn't make into the abbreviation). Neu5Ac has a crinkled ring shape with stuff hanging off of it and can attach (link) to still other sugars in two different ways, designated α and β. Remember where Neu5Ac is: at the tip of a long chain of sugar units, the other end of which connects either directly to the cell membrane (glycolipids) or to proteins that stick through the cell membrane (glycoproteins). So there are other sugars before the sialic acid connecting it to the cell surface or surface tethered protein. We will only be concerned with the next one in line when Neu5Ac is linked at its carbon #2 via an α-linkage to a sugar called galactose at its carbon #3 or #6. We designate these sugar pairs at the end of the sugar chains Neu5Ac-α2, 3Gal and Neu5Ac-α2, 6Gal. It should be understood that there will be still more sugars connected to the galactose further in, leading to lots of other possible patterns, some of which are thought to be important in one way or another.
So Part II brought us to this point: avian influenza viruses like to grab on to cells with Neu5Ac-α2, 3Gal on their surfaces, human influenza viruses like to grab on to cells with Neu5Ac-α2, 6Gal on their surfaces. When we first realized this, it seemed the picture was starting to really make sense. It got even more interesting when it was found that birds had Neu5Ac-α2, 3Gal cells in their intestines and humans had Neu5Ac-α2, 6Gal in their respiratory tracts and some animals, like pigs, had both. That made pigs a possible "mixing vessel" for both human and bird viruses. It was a great story. Unfortunately, as usually happens, the more we learn the less we know. So let's continue the story a bit further (I originally was going to make this a simple two parter that explained the Turkish mutation story but every thread I pull seems to unravel it more).
From now on I am going to refer to Neu5Ac-α2, 3Gal and its companion in shorthand by α-2, 3 and α-2, 6. The problem began a few years ago when it was discovered that human lungs have both cells with α-2, 3 on their surface and cells with α-2, 6. Bummer. It is still a bit unclear which cells in the respiratory tract have which surface markers, but it appears that the ciliated cells in the upper respiratory tract have α-2, 3 and the non-ciliated cells have α-2, 6. Ciliated? Non-ciliated? OK. We have to pause for a quick look at lung histology (the structure and cell types of the lung).
First a review of the respiratory system. Think of it as an upside down tree with two functional parts. First is the conducting system part, consisting of the root of the tree (your nose and mouth) connected to a trunk (the trachea) which branches right and left, branches again into bronchi, etc. until it gets down to very fine twigs. The conducting system, as its name implies, conducts oxygen from the outside in and waste gas (mainly carbon dioxide) from the inside out. At the ends of the twigs are the leaves, the gas exchange functional part. This is where oxygen and carbon dioxide pass back and forth between your blood and the conducting system. Here's a picture (the one I used at the Flu Wiki Influenza Primer I (go there for more explanation of influenza as a clinical illness):
You can see the upside-down tree easily here, although the gas exchange units are not indicated. They would be at the ends of the little branches if we drew them. The gas exchange units (the alveoli) are very thin, delicate structures which have to be protected from dirt, junk and microbes that might be breathed in. There is a lot going on in producing that protection, but we will only consider one aspect of it, located further up in the conducting system.
Imagine a particle, like a piece of dust that you inhale. What prevents that from getting all the way down into the gas exchange units and clogging them up? There are a lot of twists and turns as the air stream goes down the conducting system and the larger particles can't maneuver it very well. Think of a tight curvy race track. If you are driving a big truck or an SUV very fast along it it is not going to be able to make the quick turns and you will wind up crashing into the wall. Only the very small, speedy sports cars can maneuver the course. So it is with most particles you inhale. Those over ten microns in diameter will wind up smashed against one of the walls of the conducting system. You wouldn't want it to be stuck there for all time, however, because you'd eventually clog up the pipe. So your respiratory system has a way to remove them. The conducting system is lined with cells (cells on the surface of an organ are called epithelial cells, so these are bronchial or tracheal epithelial cells) and some of them secrete mucus which entraps the particles. Now we have to move the particles up and out of the conducting system and this is done by specialized cells that have little hairs sticking out into the pipe interior. The hairs, called cilia, are connected to a little cellular motor which makes them beat or wave in unison, fast in the upward direction, slow in the downward direction. The result is that the layer of mucus is slid upwards until its far end gets to the back of your throat where you either spit it out or swallow it. This apparatus has the picturesque name of "the mucociliary escalator." It turns out that one of the constituents of mucus is a substance called mucin, a large protein studded with sugars, some of have abundant α-linked 2, 3 sialic acids. Interesting. Runny nose? Coughing up mucus?
Anyway, most of the cells in the upper conducting system (nose, mouth, throat, larynx, trachea, bronchi) where the influenza virus first begins its infections are ciliated cells, but there are also some non-ciliated cells, including the mucus secreting goblet cells and some other cells of various kinds. It turns out that the ciliated cells have α-2, 3 on their surfaces while the non-ciliated ones have α-2, 6. This is just the reverse of what we would expect and the explanation is not completely clear at this time. But here's tone of the explanations that has been put forth and it makes some sense. Human influenza viruses infect initially cells that have α-2, 6 on their surface, in this case the minority of cells that are non-ciliated. But we can see that once they have infected these cells they produce such abundant virus that the heavy local dose can infect the ciliated cells that are right next to them and little foci of infected non-ciliated and ciliated cells get bigger and coalesce. When you look late in an influenza infection you see mainly infected ciliated cells (since they make up most of the cells). But it starts with the non-ciliated ones and spreads.
Well if ciliated cells can be infected by human influenza viruses even though they have α-2, 3 on their surfaces, why aren't we susceptible to bird viruses right away? We don't know the answer to this for sure, but one plausible explanation involves the mucus layer which is loaded with α-2, 3 receptors that can bind the virus and keep it from reaching the cells in any great quantity. You can overcome this safeguard with large exposures, which may be why we see human infections in those with heavy exposure to poultry. The mucus doesn't protect at all against the α-2, 6-loving human virus, so it is free to infect the non-ciliated cells and produce a high enough dose to infect them first and then the rest. But there is clearly more to the story than this, but we don't know most of what is going on here. In any event, the original α-2, 3 = birds and α-2, 6 = humans story doesn't quite do the trick. Too bad.
I still haven't gotten to the Turkish mutations (and it now turns out there were three of them). That's for a later post.
We know that viruses aren't completely promiscuous, however. They usually are restricted in the kinds of animals and the tissues or organ cells they infect. In humans, influenza is primarily (although not exclusively) a respiratory disease, at least at first. Moreover, influenza viruses that infect birds don't usually readily infect humans. We now know that influenza viruses can "jump the species barrier" and ones that formerly just infected birds can start infecting humans. Since H5N1 is primarily a bird (avian) influenza virus, and in birds usually infects the bird's intestines, the question of what allows a virus to infect particular animals and tissues is obviously an important question.
In Part I of this series we discussed what the influenza virus "sees" when it cruises by a cell, describing the lawn or canopy of sugars that cover the surface of most cells. Part I. also introduced some terms (like glycolipid and glycoprotein) you will encounter when reading papers about influenza. The main idea, however, was to give you a picture (both literally and conceptually) of where the action is taking place at the surface of a host cell. In Part II I discussed a particular sugar that tips the ends of this lawn of sugar chains sticking up from the cell's surface. It is called sialic acid. The terminology that is used in this field is keyed to the details of the chemical structure of sialic acid, so we spent most of Part II explaining that terminology. It isn't really necessary to know why something is called an α-2, 3 linkage or an α-2, 6 linkage, but I like to know these things and I thought I'd take the time to explain it to you. My (long) experience as a professor has taught me that people get pleasure out of understanding things, even if it is only vaguely. If it's not your cup of tea, just skip it.
Here's a quick summary of the gist of Part II. The particular sialic acid we are concerned with has a modification to it, an attached chemical group called an acetyl group, hooked on at the #5 carbon through a Nitrogen atom. We show the numbering and other stuff in the post. Sialic acid also has another name, neuraminic acid, so this modified sialic acid is also designated Neu5Ac (neuraminic acid N-acetylated at position 5; somehow the "N" part didn't make into the abbreviation). Neu5Ac has a crinkled ring shape with stuff hanging off of it and can attach (link) to still other sugars in two different ways, designated α and β. Remember where Neu5Ac is: at the tip of a long chain of sugar units, the other end of which connects either directly to the cell membrane (glycolipids) or to proteins that stick through the cell membrane (glycoproteins). So there are other sugars before the sialic acid connecting it to the cell surface or surface tethered protein. We will only be concerned with the next one in line when Neu5Ac is linked at its carbon #2 via an α-linkage to a sugar called galactose at its carbon #3 or #6. We designate these sugar pairs at the end of the sugar chains Neu5Ac-α2, 3Gal and Neu5Ac-α2, 6Gal. It should be understood that there will be still more sugars connected to the galactose further in, leading to lots of other possible patterns, some of which are thought to be important in one way or another.
So Part II brought us to this point: avian influenza viruses like to grab on to cells with Neu5Ac-α2, 3Gal on their surfaces, human influenza viruses like to grab on to cells with Neu5Ac-α2, 6Gal on their surfaces. When we first realized this, it seemed the picture was starting to really make sense. It got even more interesting when it was found that birds had Neu5Ac-α2, 3Gal cells in their intestines and humans had Neu5Ac-α2, 6Gal in their respiratory tracts and some animals, like pigs, had both. That made pigs a possible "mixing vessel" for both human and bird viruses. It was a great story. Unfortunately, as usually happens, the more we learn the less we know. So let's continue the story a bit further (I originally was going to make this a simple two parter that explained the Turkish mutation story but every thread I pull seems to unravel it more).
From now on I am going to refer to Neu5Ac-α2, 3Gal and its companion in shorthand by α-2, 3 and α-2, 6. The problem began a few years ago when it was discovered that human lungs have both cells with α-2, 3 on their surface and cells with α-2, 6. Bummer. It is still a bit unclear which cells in the respiratory tract have which surface markers, but it appears that the ciliated cells in the upper respiratory tract have α-2, 3 and the non-ciliated cells have α-2, 6. Ciliated? Non-ciliated? OK. We have to pause for a quick look at lung histology (the structure and cell types of the lung).
First a review of the respiratory system. Think of it as an upside down tree with two functional parts. First is the conducting system part, consisting of the root of the tree (your nose and mouth) connected to a trunk (the trachea) which branches right and left, branches again into bronchi, etc. until it gets down to very fine twigs. The conducting system, as its name implies, conducts oxygen from the outside in and waste gas (mainly carbon dioxide) from the inside out. At the ends of the twigs are the leaves, the gas exchange functional part. This is where oxygen and carbon dioxide pass back and forth between your blood and the conducting system. Here's a picture (the one I used at the Flu Wiki Influenza Primer I (go there for more explanation of influenza as a clinical illness):
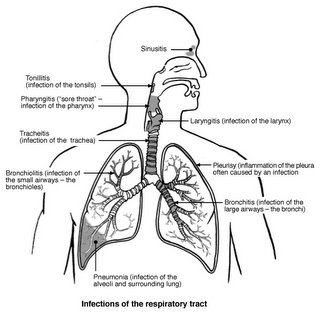
Imagine a particle, like a piece of dust that you inhale. What prevents that from getting all the way down into the gas exchange units and clogging them up? There are a lot of twists and turns as the air stream goes down the conducting system and the larger particles can't maneuver it very well. Think of a tight curvy race track. If you are driving a big truck or an SUV very fast along it it is not going to be able to make the quick turns and you will wind up crashing into the wall. Only the very small, speedy sports cars can maneuver the course. So it is with most particles you inhale. Those over ten microns in diameter will wind up smashed against one of the walls of the conducting system. You wouldn't want it to be stuck there for all time, however, because you'd eventually clog up the pipe. So your respiratory system has a way to remove them. The conducting system is lined with cells (cells on the surface of an organ are called epithelial cells, so these are bronchial or tracheal epithelial cells) and some of them secrete mucus which entraps the particles. Now we have to move the particles up and out of the conducting system and this is done by specialized cells that have little hairs sticking out into the pipe interior. The hairs, called cilia, are connected to a little cellular motor which makes them beat or wave in unison, fast in the upward direction, slow in the downward direction. The result is that the layer of mucus is slid upwards until its far end gets to the back of your throat where you either spit it out or swallow it. This apparatus has the picturesque name of "the mucociliary escalator." It turns out that one of the constituents of mucus is a substance called mucin, a large protein studded with sugars, some of have abundant α-linked 2, 3 sialic acids. Interesting. Runny nose? Coughing up mucus?
Anyway, most of the cells in the upper conducting system (nose, mouth, throat, larynx, trachea, bronchi) where the influenza virus first begins its infections are ciliated cells, but there are also some non-ciliated cells, including the mucus secreting goblet cells and some other cells of various kinds. It turns out that the ciliated cells have α-2, 3 on their surfaces while the non-ciliated ones have α-2, 6. This is just the reverse of what we would expect and the explanation is not completely clear at this time. But here's tone of the explanations that has been put forth and it makes some sense. Human influenza viruses infect initially cells that have α-2, 6 on their surface, in this case the minority of cells that are non-ciliated. But we can see that once they have infected these cells they produce such abundant virus that the heavy local dose can infect the ciliated cells that are right next to them and little foci of infected non-ciliated and ciliated cells get bigger and coalesce. When you look late in an influenza infection you see mainly infected ciliated cells (since they make up most of the cells). But it starts with the non-ciliated ones and spreads.
Well if ciliated cells can be infected by human influenza viruses even though they have α-2, 3 on their surfaces, why aren't we susceptible to bird viruses right away? We don't know the answer to this for sure, but one plausible explanation involves the mucus layer which is loaded with α-2, 3 receptors that can bind the virus and keep it from reaching the cells in any great quantity. You can overcome this safeguard with large exposures, which may be why we see human infections in those with heavy exposure to poultry. The mucus doesn't protect at all against the α-2, 6-loving human virus, so it is free to infect the non-ciliated cells and produce a high enough dose to infect them first and then the rest. But there is clearly more to the story than this, but we don't know most of what is going on here. In any event, the original α-2, 3 = birds and α-2, 6 = humans story doesn't quite do the trick. Too bad.
I still haven't gotten to the Turkish mutations (and it now turns out there were three of them). That's for a later post.
<< Home